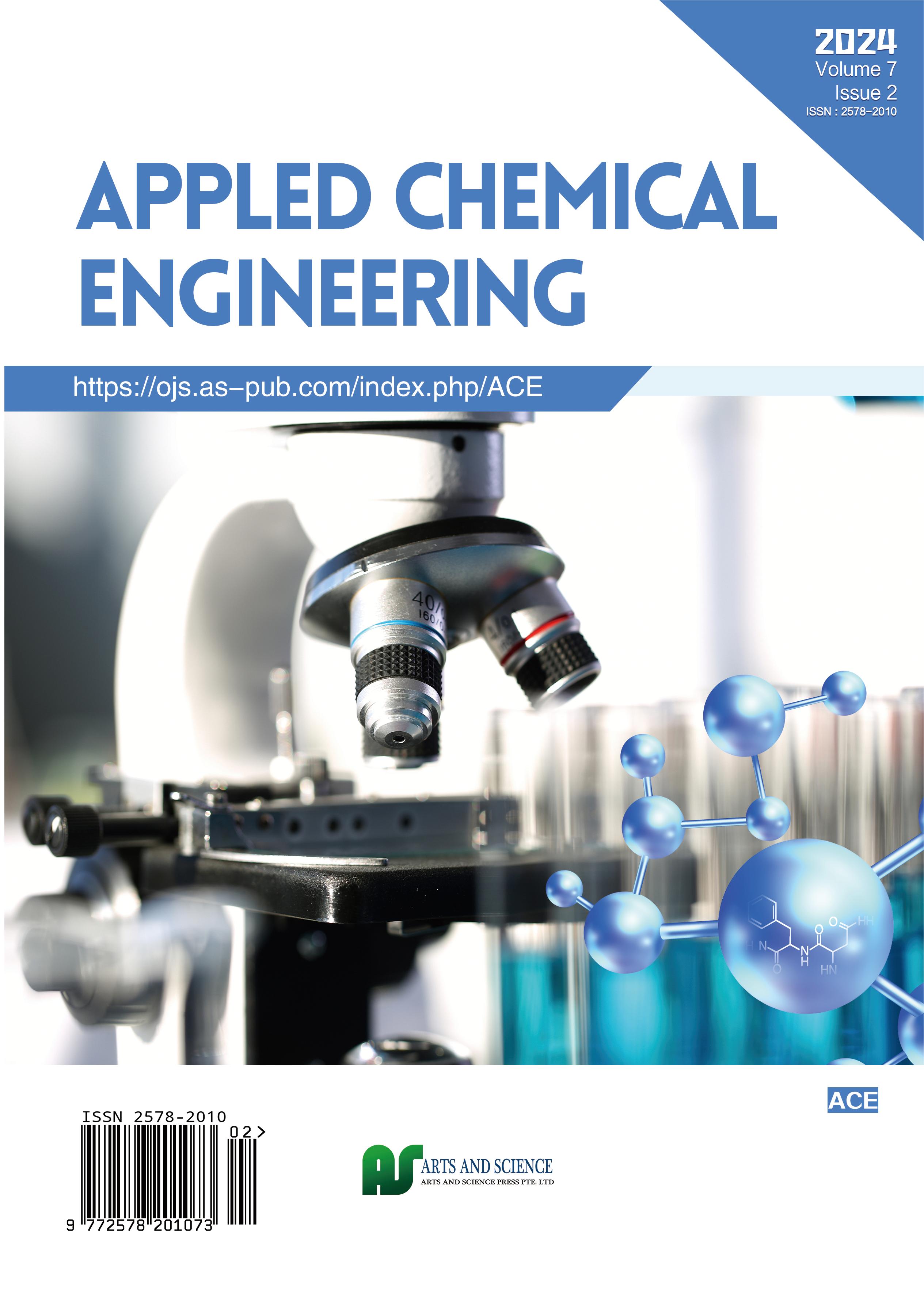
Published
2024-04-15
Issue
Section
Original Research Article
License
Copyright (c) 2024 Natalia Kamanina

This work is licensed under a Creative Commons Attribution 4.0 International License.
The Author(s) warrant that permission to publish the article has not been previously assigned elsewhere.
Author(s) shall retain the copyright of their work and grant the Journal/Publisher right for the first publication with the work simultaneously licensed under:
OA - Creative Commons Attribution-NonCommercial 4.0 International (CC BY-NC 4.0). This license allows for the copying, distribution and transmission of the work, provided the correct attribution of the original creator is stated. Adaptation and remixing are also permitted.
This license intends to facilitate free access to, as well as the unrestricted reuse of, original works of all types for non-commercial purposes.
How to Cite
Nano-structuration influence on the polyimide-based spatial light modulator’s parameters
Natalia Kamanina
St.-Petersburg Electrotechnical University (LETI)
DOI: https://doi.org/10.59429/ace.v7i2.1868
Keywords: nano-structuration; spatial light modulator; photo-layers; polyimide; liquid crystal; resolution; sensitivity; speed; fullerene doping
Abstract
The classical and nano-structured spatial light modulators (SLMs) especially based on the polyimide photosensitive layers, as the key element of the optoelectronic, display, and telecommunications schemes are considered. A modulator’s basic characteristics are studied taken into account the comparison with the different types of the photo-layers, such as: ZnSe, ZnS, a-Si:H. Liquid crystal (LC) media is considered as the modulation system. It is indicated that the different methods and approaches are applied for investigation of the basic SLM parameters, such as: Z-scanning technique, third harmonic generation, four-wave mixing set-up, etc. In the current paper the laser holographic technique is used to investigate the resolution, sensitivity, and speed of the LC-SLM devices. As the main aspect, the influence of the fullerene doping on the organic photo-layers based on the polyimide materials is presented. This influence of this nano-structuration process on the modulator’s basic parameters is discussed.
References
[1]. Petrov MP, Stepanov SI, Khomenko AV. Photosensitive Electrooptical Media for Holography and Optical Information Processing (Russian). Nauka; 1983. 268p.
[2]. Hauck R, Bryngdahl O. Computer-generated holograms with pulse-density modulation. Journal of the Optical Society of America A 1984; 1(1): 5–10. doi: 10.1364/JOSAA.1.000005
[3]. Vasil'ev AA, Casasent D, Kompanets LN, et al. Spatial Light Modulators (Russian). Radio i Svyaz'; 1987. 320p.
[4]. Groznov MA, Kamanina NV, Myl’nikov MS, et al. Optical homogeneity of light modulators of the chalcogenide vitreous semiconductor—Liquid crystal type. Soviet Journal of Optical Technology 1989; 56(5): 267–268.
[5]. Dumarevskii YuD, Zakharova TV, Kovtonyuk NF, et al. Characteristics of liquid-crystal optically controlled transparencies based on a-CdTe and a-Si:H photosensitive layers. Soviet Journal of Optical Technology 1989; 56: 729–732.
[6]. Kamanina NV, Soms LN, Tarasov AA. Correction of phase aberrations by the holographic method using liquid-crystal spatial light modulators. Optics and Spectroscopy 1990; 68(3): 403–404.
[7]. Godya SI, Danilyuk SA, Ermakov AS, et al. Liquid crystal space-time light modulators with thin zinc selenide films. Soviet Physics Technical Physics 1991; 61: 349–351.
[8]. Aleksandrov BG, Nikitin VV, Kuz'mina II, et al. Measurement of the dynamic characteristics of optically addressed spatial light modulators. Soviet Journal of Optical Technology 1992; 59: 75–77.
[9]. Vladimirov FL, Chaika AN, Morichev IE, et al. Temporal characteristics of optically controlled transparencies of the photoconductor—Liquid crystal type used as holographic correlators. Soviet Journal of Optical Technology 1993; 60: 483–486.
[10]. Ivanova NL, Kamanina NV, Komarov AP, et al. Optimization of the dynamic characteristics of space-time light modulators with an a-Si:H photolayer. Technical Physics Letters 1993; 19(4): 216–218.
[11]. Belyaev VV, Chigrinov VG. Figure of merit of liquid crystal materials for optically addressed spatial modulators. Applied Optics 1993; 32(2): 141–146. doi: 10.1364/AO.32.000141
[12]. Mylnikov VS. Photoconducting Polymers. Springer-Verlag; 1994. 88p.
[13]. Kamanina NV. Using the operator Laplace method to estimate the response time of space-time light modulators. Technical Physics 1994; 39(1): 83–85.
[14]. Blinov LM, Chigrinov VG. Electrooptic Effects in Liquid Crystal Materials. Springer; 1994. 459p.
[15]. Kamanina NV, Vasilenko NA. Effect of various alignment films on dynamic characteristics of LC spatial light modulators. Proceedings of SPIE 1996; 2731: 220–226. doi: 10.1117/12.230665
[16]. Meier U, Bösch M, Bosshard C, Günter P. DAST a high optical nonlinearity organic crystal. Synthetic Metals 2000; 109(1–3): 19–22. doi: 10.1016/S0379-6779(99)00190-3
[17]. Coomber SD, Cameron CD, Hughes JR, et al. Optically addressed spatial light modulators for replaying computer-generated holograms. In: Proceedings Volume 4457, Spatial Light Modulators: Technology and Applications; 8 November 2001; San Diego, CA, United States. Volume 4457. pp. 9–19.
[18]. Follonier S, Fierz M, Biaggio I, et al. Structural, optical, and electrical properties of the organic molecular crystal 4-N,N-dimethylamino-4’-N’-methyl stilbazolium tosylate. Journal of the Optical Society of America B 2002; 19(9): 1990–1998. doi: 10.1364/JOSAB.19.001990
[19]. Birch P, Young R, Chatwin C, et al. Fully complex optical modulation with an analogue ferroelectric liquid crystal spatial light modulator. Optics Communications 2000; 175(4–6): 347–352. doi: 10.1016/S0030-4018(00)00478-8
[20]. Jesacher A, Schwaighofer A, Fürhapter S, et al. Wavefront correction of spatial light modulators using an optical vortex image. Optics Express 2007; 15(9): 5801–5808. doi: 10.1364/OE.15.005801
[21]. Kelemen L, Ormos P, Vizsnyiczai G. Two-photon polymerization with optimized spatial light modulator. Journal of the European Optical Society 2011; 6: 11029. doi: 10.2971/jeos.2011.11029
[22]. Fan F, Du T, Srivastava AK, et al. Axially symmetric polarization converter made of patterned liquid crystal quarter wave plate. Optics Express 2012; 21(20): 23036–23043. doi: 10.1364/OE.20.023036
[23]. Huang SY, Zheng HY, Yu KY, et al. Electrically tunable prism grating based on a liquid crystal film with a photoconductive layer. Optical Materials Express 2012; 2(12): 1791–1796. doi: 10.1364/OME.2.001791
[24]. Sun J, Chen Y and Wu ST. Submillisecond-response and scattering-free infrared liquid crystal phase modulators. Optics Express 2012; 20(18): 20124–20129. doi: 10.1364/OE.20.020124
[25]. Song Z, Yu Y, Zhang X, et al. Optical microfiber phase modulator directly driven with low-power light. Chinese Optics Letters 2014; 12(9): 090606. doi: 10.3788/COL201412.090606
[26]. Cai L, Mahmoud A, Khan M, et al. Acousto-optical modulation of thin film lithium niobate waveguide devices. Photonics Research 2019; 7(9): 1003–1013. doi: 10.1364/PRJ.7.001003
[27]. Uthayakumar M, Saraswathi V, Pasupathi G, et al. Structural, third-order nonlinear optical properties and optical limiting studies of novel zinc potassium aluminum sulfate nonadecahydrate single crystal. Journal of Materials Science: Materials in Electronics 2020; 31: 22522–22533. doi: 10.1007/s10854-020-04763-z
[28]. Jeyaram S. Intermolecular charge transfer in donor—Acceptor substituted triarylmethane dye for NLO and optical limiting applications. Journal of Materials Science: Materials in Electronics 2021; 32: 9368–9376. doi: 10.1007/s10854-021-05600-7
[29]. Jajszczyk A. Optical networks—The electro-optic reality. Optical Switching and Networking 2005; 1(1): 3–18. doi: 10.1016/j.osn.2004.11.002
[30]. van Putten EG, Vellekoop IM, Mosk AP. Spatial amplitude and phase modulation using commercial twisted nematic LCDs. Applied Optics 2008; 47(12): 2076–2081. doi: 10.1364/AO.47.002076
[31]. Sun Z, Zheng Y, Fu Y. Graphene-based spatial light modulator using metal hot spots. Materials 2019; 12(19): 3082. doi: 10.3390/ma12193082
[32]. Hsu WF, Weng MH. Compact holographic projection display using liquid-crystal-on-silicon spatial light modulator. Materials 2016; 9(9): 768. doi: 10.3390/ma9090768
[33]. Mahafzah BA, Al-Adwan AA, Zaghloul RI. Topological properties assessment of optoelectronic architectures. Telecommunication Systems 2022; 80(4): 599–627. doi: 10.1007/s11235-022-00910-5
[34]. Kozacki T, Chlipala M, Martinez-Carranza J, et al. LED near-eye holographic display with a large non-paraxial hologram generation. Optics Express 2022; 30(24): 43551–4365. doi: 10.1364/OE.468823
[35]. Seki A, Shimizu K, Aoki K. Chiral π-conjugated liquid crystals: Impacts of ethynyl linker and bilateral symmetry on the molecular packing and functions. Crystals 2022; 12(9): 1278. doi: 10.3390/cryst12091278
[36]. Kamanina NV. Electrooptical systems based on the liquid crystals and fullerenes—Perspective materials of the nanoelectronics. Properties and area of the applications. Textbook for masters. IFMO University Press; 2008. 136p.
[37]. Heo J, Huh JW, Yoon TH. Fast-switching initially-transparent liquid crystal light shutter with crossed patterned electrodes. AIP Advances 2015; 5: 047118. doi: 10.1063/1.4918277
[38]. Lin GJ, Chen TJ, Lin YT, et al. Effects of chiral dopant on electro-optical properties of nematic liquid crystal cells under in-plane switching and non-uniform vertical electric fields. Optical Materials Express 2014; 4(12): 2468–2477. doi: 10.1364/OME.4.002468
[39]. Macfaden AJ, Wilkinson TD. Characterization, design, and optimization of a two-pass twisted nematic liquid crystal spatial light modulator system for arbitrary complex modulation. Journal of the Optical Society of America A 2017; 34(2): 161–170. doi: 10.1364/JOSAA.34.000161
[40]. Clark NA, Lagerwall ST. Submicrosecond bistable electro‐optic switching in liquid crystals. Applied Physics Letters 1980; 36(11): 899–901. doi: 10.1063/1.91359
[41]. Wahle M, Kasdorf O, Kitzerow HS, et al. Electrooptic switching in graphene-based liquid crystal cells. Molecular Crystals and Liquid Crystals 2011; 543(1): 187–193. doi: 10.1080/15421406.2011.569524
[42]. Honma M, Miura M, Nose T. Liquid-crystal-grating-based optical displacement sensors. Applied Optics 2016; 55(35): 10045–10052. doi: 10.1364/AO.55.010045
[43]. Likhomanova SV, Kamanina NV. COANP-fullerenes system for optical modulation. Journal of Physics: Conference Series 2016; 741: 012146. doi: 10.1088/1742-6596/741/1/012146
[44]. Belyaev VV, Solomatin AS, Chausov DN, et al. optical properties of composite heterophase objects with liquid crystal material for different display applications. Journal of the Society for Information Display 2017; 25(9): 561–567. doi: 10.1002/jsid.606
[45]. Kamanina NV. Nanoparticles doping influence on the organics surface relief. Journal of Molecular Liquids 2019; 283: 65–68. doi: 10.1016/j.molliq.2019.03.043
[46]. Kamanina NV, Toikka AS, Zvereva GN, et al. Surface relief of polyimide thin-film orienting materials for liquid crystalline light modulators (Russian). Liquid Crystals and their Application 2021; 21(4): 47–52. doi: 10.18083/LCAppl.2021.4.47
[47]. Kamanina NV. Similarities and differences between the effect of orientation of red blood cells in a nematic liquid-crystal medium and the Fröhlich electrical vibrations. Technical Physics Letters 1997; 23(12): 902–905. doi: 10.1134/1.1261926
[48]. Kamanin AA, Kamanina NV. Erythrocyte induced structurization of a liquid crystal mesophase. Technical Physics Letters 2006; 32(7): 610–613. doi: 10.1134/S1063785006070182
[49]. Kamanina NV, Vasilenko NA. Influence of operating conditions and of interface properties on dynamic characteristics of liquid-crystal spatial light modulators. Optical and Quantum Electronics 1997; 29(1): 1–9. doi: 10.1023/A:1018506528934
[50]. Kamanina NV. Mechanisms of optical limiting in -conjugated organic system: Fullerene-doped polyimide. Synthetic Metals 2022; 127(1–3): 121–128. doi: 10.1016/S0379-6779(01)00598-7
[51]. Kamanina NV, Uskokovic DP. Refractive index of organic systems doped with nano-objects. Materials and Manufacturing Processes 2008; 23(6): 552–556. doi: 10.1080/10426910802157722
[52]. Kamanina NV, Emandi A, Kajzar F, et al. Laser-induced change in the refractive index in the systems based on nanostructured polyimide: Comparative study with other photosensitive structures. Molecular Crystals and Liquid Crystals 2008; 486(1): 1–11. doi: 10.1080/15421400801914319
[53]. Kamanina NV. The effect of the charge transfer pathway during intermolecular complex formation on nonlinear optical and photoconducting properties of nanocomposites. Technical Physics Letters 2012; 38(2): 114–117. doi: 10.1134/S1063785012020083
[54]. Gutman F, Lyons LE. Organic Semiconductors (Russian). John Wileys & Sons; 1967. 858p.
[55]. Rout S, Sonkusale S. Wireless multi-level terahertz amplitude modulator using active metamaterial-based spatial light modulation. Optics Express 2016; 24(13): 14618–14631. doi: 10.1364/OE.24.014618
[56]. Zhang Y, Ren Y, Chen J, et al. Fast testing of partial camera lenses based on a liquid crystal spatial light modulator. Applied Optics 2022; 61(22): 6420–6429. doi: 10.1364/AO.460384
[57]. Alaloul M, Khurgin JB, Al-Ani I, et al. On-chip low-loss all-optical MoSe2 modulator. Optics Letters 2022; 47(15): 3640–3643. doi: 10.1364/OL.465171
[58]. Shcherbin K, Gvozdovskyy I, Shumelyuk A, et al. Near-infrared sensitive two-wave mixing adaptive interferometer based on a liquid crystal light valve with a semiconductor substrate. Applied Optics 2022; 61(22): 6498–6503. doi: 10.1364/AO.465085
[59]. Du Q, Ai J, Qin Z, et al. Fabrication of superhydrophobic/superhydrophilic patterns on polyimide surface by ultraviolet laser direct texturing. Journal of Materials Processing Technology 2018; 251: 188–196. doi: 10.1016/j.jmatprotec.2017.08.034
[60]. Cherkashina NI, Pavlenko VI, Abrosimov VM, et al. Effect of 10 MeV electron irradiation on polyimide composites for space systems. Acta Astronautica 2021; 184: 59–69. doi: 10.1016/j.actaastro.2021.03.032
[61]. Sorianello V, Midrio M, Contestabile G, et al. Graphene—Silicon phase modulators with gigahertz bandwidth. Nature Photonics 2018; 12(1): 40–44. doi: 10.1038/s41566-017-0071-6
[62]. Gan X, Zhao C, Wang Y, et al. Graphene-assisted all-fiber phase shifter and switching. Optica 2015; 2(5): 468–471. doi: 10.1364/OPTICA.2.000468
[63]. Wang Y, Gan X, Zhao C, et al. All-optical control of microfiber resonator by graphene’s photothermal effect. Applied Physics Letters 2016; 108(17): 171905. doi: 10.1063/1.4947577
[64]. Zhong C, Li J, Lin H. Graphene-based all-optical modulators. Frontiers of Optoelectronics 2020; 13(2): 114–128. doi: 10.1007/s12200-020-1020-4
[65]. Zhang X, Zhang B, Sun M, et al. Preparation and thermal conductivity properties of high-temperature resistance polyimide composite films based on silver nanowires-decorated multi-walled carbon nanotubes. Journal of Materials Science: Materials in Electronics 2022; 33: 1577–1588. doi: 10.1007/s10854-021-07680-x
[66]. Chan CH, Wu TY, Yen MH, et al. Low power consumption and high-contrast light scattering based on polymer-dispersed liquid crystals doped with silver-coated polystyrene microspheres. Optics Express 2016; 24(26): 29963–29971. doi: 10.1364/OE.24.029963
[67]. Kim H, Inoue Y, Kobashi J, et al. Deformation-free switching of polymer-stabilized cholesteric liquid crystals by low temperature polymerization. Optical Materials Express 2016; 6(3): 705–710. doi: 10.1364/OME.6.000705