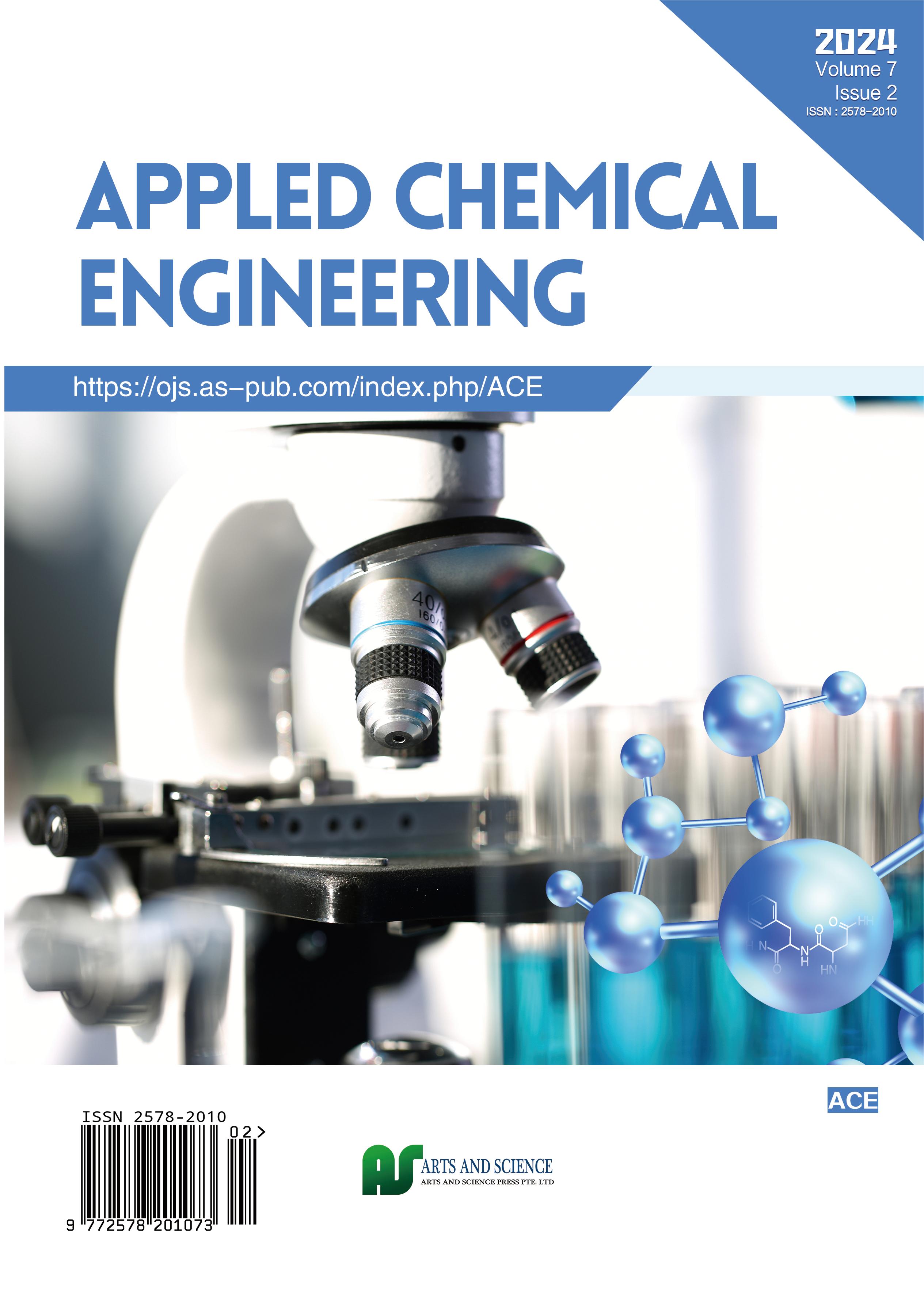
Published
2024-04-15
Issue
Section
Original Research Article
License
Copyright (c) 2024 yilmaz yurekli, Sadika Guedidi Bernard, Sacide Alsoy Altinkaya, André Deratani, Christophe Innocent

This work is licensed under a Creative Commons Attribution 4.0 International License.
The Author(s) warrant that permission to publish the article has not been previously assigned elsewhere.
Author(s) shall retain the copyright of their work and grant the Journal/Publisher right for the first publication with the work simultaneously licensed under:
OA - Creative Commons Attribution-NonCommercial 4.0 International (CC BY-NC 4.0). This license allows for the copying, distribution and transmission of the work, provided the correct attribution of the original creator is stated. Adaptation and remixing are also permitted.
This license intends to facilitate free access to, as well as the unrestricted reuse of, original works of all types for non-commercial purposes.
How to Cite
Behavior of enzymatic membranes under pressure: Effect of enzyme location
Yilmaz Yurekli
Bioengineering Department, Manisa Celal Bayar University
Sadika Guedidi
European Membrane Institute, Montpellier 2 University (ENSCM, UM2, CNRS)
Sacide Alsoy Altinkaya
Department of Chemical Engineering, Izmir Institute of Technology
André Deratani
European Membrane Institute, Montpellier 2 University (ENSCM, UM2, CNRS)
Christophe Innocent
European Membrane Institute, Montpellier 2 University (ENSCM, UM2, CNRS)
DOI: https://doi.org/10.59429/ace.v7i2.2064
Keywords: enzymatic membrane; LbL self assembly; mass transfer resistance; long term stability; trypsin; urease
Abstract
Enzyme immobilized membranes combine catalysis and separation functions. Their application in large-scale continuous processes requires knowing the behavior under pressure. Also, the effects of enzyme location on the mass transfer limitation, membranes’ stability, and filtration performance should be investigated. In this study, urease (URE) and trypsin (TRY) enzymes were physically immobilized in/on the surface of a polyacrylonitrile (AN69) membrane through layer-by-layer (LbL) self-assembly method using polyethylenemine (PEI) and sodium-alginate (ALG) as cationic and anionic polyelectrolytes respectively. URE, located on the membrane’s surface, degraded urea in a reaction-controlled regime, and its immobilization did not significantly change the hydraulic permeability. On the other hand, the TRY enzyme attached to the membrane’s pores reduced the permeability and degraded the BAPNA in a diffusion-controlled region. In TRY immobilized membranes, the conversion increased linearly with the transmembrane pressure, while in URE immobilized ones, conversion was maximum at 1 bar. Sandwiching the enzymes between two polyelectrolytes resulted in the highest catalytic activities. This configuration maintained most of the URE activity in the long-term filtration, but it did not help prevent TRY’s activity loss.
Author Biographies
Yilmaz Yurekli, Bioengineering Department, Manisa Celal Bayar University
Bioengineering Department
Sadika Guedidi, European Membrane Institute, Montpellier 2 University (ENSCM, UM2, CNRS)
Institut Européen des Membranes
Sacide Alsoy Altinkaya, Department of Chemical Engineering, Izmir Institute of Technology
Chemical Engineering Department
André Deratani, European Membrane Institute, Montpellier 2 University (ENSCM, UM2, CNRS)
Institut Européen des Membranes
Christophe Innocent, European Membrane Institute, Montpellier 2 University (ENSCM, UM2, CNRS)
Institut Européen des Membranes
References
[1]. Inamdar STA. Biochemical Engineering: Principles and Concepts, 3rd ed. PHI; 2012.
[2]. Rodrigues RC, Ortiz C, Berenguer-Murcia Á, et al. Modifying enzyme activity and selectivity by immobilization. Chemical Society Reviews 2013; 42(15): 6290–6307. doi: 10.1039/c2cs35231a
[3]. Bayne L, Ulijn RV, Halling PJ. Effect of pore size on the performance of immobilised enzymes. Chemical Society Reviews 2013; 42(23): 9000. doi: 10.1039/c3cs60270b
[4]. Liu Y, Lu H, Zhong W, et al. Multilayer-assembled microchip for enzyme ımmobilization as reactor toward low-level protein ıdentification. Analytical Chemistry 2005; 78(3): 801–808. doi: 10.1021/ac051463w
[5]. Yong JKJ, Cui J, Cho KL, et al. Surface engineering of polypropylene membranes with carbonic anhydrase-loaded mesoporous silica nanoparticles for ımproved carbon dioxide hydration. Langmuir 2015; 31(22): 6211−6219. doi: 10.1021/acs.langmuir.5b01020
[6]. Dizge N, Epsztein R, Cheng W, et al. Biocatalytic and salt selective multilayer polyelectrolyte nanofiltration membrane. Journal of Membrane Science 2018; 549: 357–365. doi: 10.1016/j.memsci.2017.12.026
[7]. Datta S, Cecil C, Bhattacharyya D. Functionalized membranes by layer-by-layer assembly of polyelectrolytes and ın situ polymerization of acrylic acid for applications in enzymatic catalysis. Industrial & Engineering Chemistry Research 2008; 47(14): 4586–4597. doi: 10.1021/ie800142d
[8]. Sarma R, Islam MdS, Miller AF, Bhattacharyya D. Layer-by-layer-assembled laccase enzyme on stimuli-responsive membranes for chloro-organics degradation. ACS Applied Materials & Interfaces 2017; 9(17): 14858−14867. doi: 10.1021/acsami.7b01999
[9]. Dong J, Ning W, Liu W, Bruening ML. Limited proteolysis in porous membrane reactors containing ımmobilized trypsin. Analyst 2017; 142(14): 2578–2586. doi: 10.1039/c7an00778g
[10]. Guedidi S, Yurekli Y, Deratani A, et al. Effect of enzyme location on activity and stability of trypsin and urease immobilized on porous membranes by using layer-by-layer self-assembly of polyelectrolyte. Journal of Membrane Science 2010; 365(1–2): 59–67. doi: 10.1016/j.memsci.2010.08.042
[11]. Yurekli Y, Alsoy SA. Catalytic performances of chemically immobilized urease under static and dynamic conditions: A comparative study. Journal of Molecular Catalysis B: Enzymatic 2011; 71(1–2): 36–44. doi: 10.1016/j.molcatb.2011.03.006
[12]. Langsdorf LJ, Zydney AL. Diffusive and convective transport through hemodialysis membranes: Comparison with hydrodynamic predictions. Journal of Biomedical Materials Research 1994; 28: 573–582. doi: 10.1002/jbm.820280507
[13]. Bradford MM. A rapid and sensitive method for the quantitation of microgram quantities of protein utilizing the principle of protein-dye binding. Analytical Biochemistry 1976; 72: 248–254. doi: 10.1006/abio.1976.9999
[14]. Smith KA, Colton CK, Merril EW, Evans LB. The artificial Kidney; Chemical Engineering Progress Symposium Series 1968; 64: 45.
[15]. Deen WM. Hindered transport of large molecules in liquid‐filled pores. AIChE Journal 1987; 33(9): 1409–1425. doi: 10.1002/aic.690330902
[16]. Bowen WR, Mohammad AW, Hilal N. Characterisation of nanofiltration membranes for predictive purposes—use of salts, uncharged solutes and atomic force microscopy. Journal of Membrane Science 1997; 126(1): 91–105. doi: 10.1016/S0376-7388(96)00276-1
[17]. Mika AM, Childs RF, Dickson JM, et al. A new class of polyelectrolyte-filled microfiltration membranes with environmentally controlled porosity. Journal of Membrane Science 1995; 108(1–2): 37–56. doi: 10.1016/0376-7388(95)00140-2
[18]. Marshall AD, Munro PA, Trägardh G. The effect of protein fouling in microfiltration and ultrafiltration on permeate flux, protein retention and selectivity: A literature review. Desalination 1993; 91(1): 65–108. doi: 10.1016/0011-9164(93)80047-Q
[19]. Musale DA, Kulkarni SS. Effect of membrane-solute interactions on ultrafiltration performance. Journal of Macromolecular Science, Part C: Polymer Reviews 1998; 38(4): 615–636. doi: 10.1080/15583729808546034
[20]. Naitou A, Oinuma M, Ozawa K, et al. Effects of charge density on electrolyte transport through dialysis membranes. Japanese Society for Artificial Organs 1987; 16(2): 703–706. doi: 10.11392/jsao1972.16.703
[21]. Koutsopoulos S, Patzsch K, Bosker WTE, Norde W. Adsorption of trypsin on hydrophilic and hydrophobic surfaces. Langmuir 2007; 23(4): 2000–2006. doi: 10.1021/la062238s
[22]. Chen PC, Ma Z, Zhu XY, et al. Fabrication and optimization of a lipase ımmobilized enzymatic membrane bioreactor based on polysulfone gradient-pore hollow fiber membrane. Catalysts 2019; 9(6): 495. doi: 10.3390/catal9060495