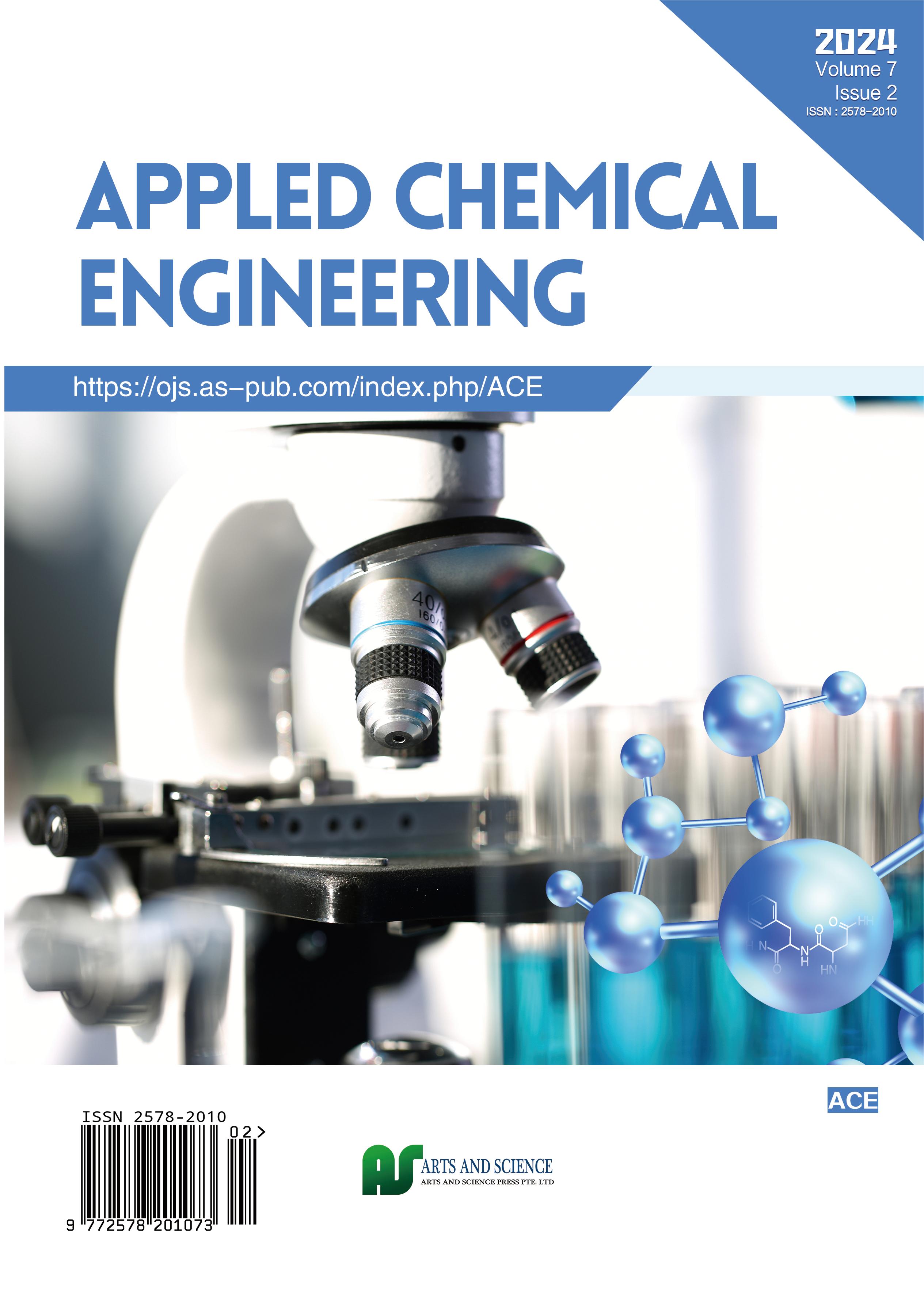
Published
2024-04-15
Issue
Section
Review Article
License
Copyright (c) 2024 S.K Osipov., V.P Sokolov., I.A Milyukov., A.N Vegera., M.M Shaikh.

This work is licensed under a Creative Commons Attribution 4.0 International License.
The Author(s) warrant that permission to publish the article has not been previously assigned elsewhere.
Author(s) shall retain the copyright of their work and grant the Journal/Publisher right for the first publication with the work simultaneously licensed under:
OA - Creative Commons Attribution-NonCommercial 4.0 International (CC BY-NC 4.0). This license allows for the copying, distribution and transmission of the work, provided the correct attribution of the original creator is stated. Adaptation and remixing are also permitted.
This license intends to facilitate free access to, as well as the unrestricted reuse of, original works of all types for non-commercial purposes.
How to Cite
A review on comparative analysis of different types of burners for oxy-fuel SCO2 recirculation and air-fuel combustion systems
S. K Osipov.
Department of Innovative Technologies for High-Tech Industries, National Research University "Moscow Power Engineering Institute"
V. P Sokolov.
Department of Innovative Technologies for High-Tech Industries, National Research University "Moscow Power Engineering Institute"
I. A Milyukov.
Department of Innovative Technologies for High-Tech Industries, National Research University "Moscow Power Engineering Institute"
A. N Vegera.
Department of Innovative Technologies for High-Tech Industries, National Research University "Moscow Power Engineering Institute"
M. M Shaikh.
Department of Innovative Technologies for High-Tech Industries, National Research University "Moscow Power Engineering Institute"
DOI: https://doi.org/10.59429/ace.v7i2.2089
Keywords: combustion system; burners; SCO2; flue gas recirculation; comparative analysis of burners
Abstract
Due to the global warming and strict environmental regulations encourages researchers to develop efficient combustion system that produce low level of harmful gases. This article focuses on review of different studies and experiment carried out in the sphere of oxy-fuel and air fuel combustion. In order to compare the results of oxy-fuel and air fuel combustion and find a suitable and efficient combustion system.
References
[1]. Pilavachi PA (2000) Power generation with gas turbine systems and combined heat and power. Appl Therm Eng 20(15):1421–1429
[2]. Angelino G. Carbon dioxide condensation cycles for power production. J Eng Gas Turbines Power 1968;90:287e95. https://doi.org/10.1115/1.3609190.
[3]. Feher EG. The supercritical thermodynamic power cycle. Energy Convers 1968;8:85e90. https://doi.org/10.1016/0013-7480(68)90105-8.
[4]. Dostal V, Driscoll MJ, Hejzlar P. A supercritical carbon dioxide cycle for next generation nuclear reactors. 2004. https://doi.org/MIT-ANP-TR-100.
[5]. Tracking IEA. Clean energy progress 2015. Paris: IEA; 2015. https://doi.org/ https://www.iea.org/reports/tracking-clean-energy-progress-2015.
[6]. Allam RJ, Fetvedt JE, Forrest BA, Freed DA. The OXY-fuel, supercritical CO2 allam cycle: new cycle developments to produce even lower-cost electricity from fossil fuels without atmospheric emissions. Proc. ASME Turbo Expo 2014. https://doi.org/10.1115/GT2014-26952
[7]. Kindra V, Rogalev A, Oparin M, Kovalev D, Ostrovsky M. Research and Development of the Oxy-Fuel Combustion Power Cycle for the Combined Production of Electricity and Hydrogen. Energies. 2023; 16(16):5983. https://doi.org/10.3390/en16165983
[8]. Rogalev N, Rogalev A, Kindra V, Zlyvko O, Bryzgunov P. Review of Closed SCO2 and Semi-Closed Oxy–Fuel Combustion Power Cycles for Multi-Scale Power Generation in Terms of Energy, Ecology and Economic Efficiency. Energies. 2022; 15(23):9226. https://doi.org/10.3390/en15239226
[9]. Burchhardt, U., Giering, R., Weiß, G., 2013. Overview of Burner Tests in Vattenfall’s Oxy-fuel Pilot Plant. In: 3rd Oxy-fuel Combustion Conference (OCC3), September 2013, Ponferrada, Spain.
[10]. Marcano, N., Recourt, P., Tsiava, R., Laurent, J., Lethier, S., Deveaux, M., Bouvarel, A., Quet, J.P., 2011. Oxy-combustion at Lacq CCS pilot plant: preliminary analysis.
[11]. Kluger, F., Mönckert, P., Bäck, A., Wang, W., Grubbström, J., Levasseur, A., Strand,M., Ecke, H., Yan, J., Burchhardt, U., 2011. Oxy-Combustion Testing in 30MWth Pilot Plant Schwarze Pumpe. In: 2nd Oxy-fuel Combustion Conference (OCC2), September 2011, Yeppoon, Australia.
[12]. Marion, J.L., Brautsch, A., Kluger, F., Larsson,M., Pourchot, T., 2011. Alstom’s Overview of a Manufacturer’s Efforts to Commercialize Oxy-Combustion for Steam Power Plants. In: 2nd Oxy-fuel Combustion Conference (OCC2), September 2011, Yeppoon, Australia.
[13]. Sturgeon, D., 2011. OxyCoalTM Burner Technology Development. In: 2nd Oxy-fuel Combustion Conference (OCC2), September 2011, Yeppoon, Australia.
[14]. Sturgeon, D.W., Rogerson, J.W., Hesselmann, G.J., 2013. OxyCoalTM Burner Testing to Develop Models for Oxy-fuel Combustion. In: 3rd Oxy-fuel Combustion Conference (OCC3), September 2013, Ponferrada, Spain.
[15]. Al-Makhadmeh, L., Maier, J., Scheffknecht, G., 2009. Coal pyrlysis and char combustion under oxy-fuel conditions. In: The Proceedings of the 34th International Technical Conference on Clean Coal & Fuel Systems, 31/05–04/06/2009, Clearwater, Florida, USA, pp. 112–123.
[16]. Rathnam, R.K., Elliott, L., Moghtaderi, B., Gupta, R., Wall, T.F., 2006. Differences in coal reactivity in air and oxy-fuel conditions and implications for coal burnout. In: Proceedings of the 31st International Technical Conference on Clean Coal & Fuel Systems, 21–26/05/2006, Clearwater, Florida, USA.
[17]. Zeng, D., Hu, S., Sarv, H., 2008. Differences in Chars Formed from Coal Pyrolysis under N2 and CO2 Atmospheres, Pittsburgh. In: Proceedings of the International Pittsburgh Coal Conference, Sept-Oct 2008, Pittsburgh, PA, USA.
[18]. Liu, H., Zailani, R., Gibbs, B.M., 2005. Pulverized coal combustion in air and in O2/CO2 mixtures with NOx recycle. Fuel 84 (16), 2109–2115, http://dx.doi.org/10.1016/j.fuel.2005.04.028
[19]. Kiga, T., Takano, S., Kimura, N., Omata, K., Okawa, M., Mori, T., Kato, M., 1997. Characteristics of pulverized-coal combustion in the system of oxygen/recycled flue gas combustion. Proc. Third Int. Conf. Carbon Dioxide Remov. 38 (Supplement 0), S129, http://dx.doi.org/10.1016/S0196-8904(96)00258-0
[20]. Molina, A., Shaddix, C., 2007a. Ignition and devolatilization of pulverized bituminous coal particles during oxygen/carbon dioxide coal combustion. Proc. Combust. Inst. 31 (2), 1905–1912, http://dx.doi.org/10.1016/j.proci.2006.08.102
[21]. Zhang, J., Kelly, K.E., Eddings, E.G., Wendt, J.O.L., 2010. Ignition in 40 kW co-axial turbulent diffusion oxy-coal jet flames. In: Proceedings of the Combustion Institute, pp. 3415–3421.
[22]. Zhang, L., Jiao, F., Binner, E., Bhattacharya, S., Ninomiya, Y., Li, C.-Z., 2011b. Experimental investigation of the combustion of bituminous coal in air and O2/CO2 mixtures: 2. Variation of the transformation behaviour of mineral matter with bulk gas composition. Fuel 90 (4), 1361–1369, http://dx.doi.org/10.1016/j.fuel. (2011).01.012
[23]. Grathwohl, S., Maier, J., Scheffknecht, G., 2011. Testing and Evaluation of Advanced Oxy-fuel Burner and Firing Concepts. In: 2nd Oxy-fuel Combustion Conference (OCC2), September 2011, Yeppoon, Australia.
[24]. Woycenko, D.M., Ikeda, I., Roberts, P., 2005. Combustion of Pulverised Coal in a Mixture of Oxygen and Recycled Flue Gas.
[25]. Payne, R., Chen, S., Wolsky, A.M., Richter, W.F., 1989. CO2 recovery via coal combustion in mixtures of oxygen and recycled flue gas. Combust. Sci. Technol. 67 (1–3), 1–16, http://dx.doi.org/10.1080/00102208908924058
[26]. Andersson, K., Johansson, R., Hjärtstam, S., Johnsson, F., Leckner, B., 2008a. Radiation intensity of lignite-fired oxy-fuel flames. Exp. Therm. Fluid Sci. 33 (1), 67–76, http://dx.doi.org/10.1016/j.expthermflusci.2008.07.010
[27]. Chen, L., Yong, S.Z., Ghoniem, A.F., 2012. Oxy-fuel combustion of pulverized coal: characterization, fundamentals, stabilization and CFD modeling. Prog. Energy Combust. Sci. 38 (2), 156–214, http://dx.doi.org/10.1016/j.pecs.(2011).09.003
[28]. Viskanta, R., 2005. Radiative Transfer of Combustion Systems: Fundamentals and Applications. Begell House, New York, xvii, 454
[29]. Andersson, K., Johansson, R., Johnsson, F., Leckner, B., 2008b. Radiation intensity of propane-fired oxy-fuel flames: implications for soot formation. Energy Fuels 22 (3), 1535–1541, http://dx.doi.org/10.1021/ef7004942
[30]. Johansson, R., Leckner, B., Andersson, K., Johnsson, F., 2013. Influence of particle and gas radiation in oxy-fuel combustion. Int. J. Heat Mass Transf. 65, 143–152, http:// dx.doi.org/10.1016/j.ijheatmasstransfer.2013.05.073
[31]. Andersson, K., Johansson, R., Johnsson, F., 2011. Modelling of particle radiation in oxy-fuel flames. In: 2nd Oxy-fuel Combustion Conference (OCC2), September 2011, Yeppoon, Australia.
[32]. Kull, R., Stein-Brzozowska, G., Theye, T., Maier, J., Scheffknecht, G., 2009. Corrosion of Superheater Materials Under Oxy-fuel Conditions. In: 1st Oxy-fuel Combustion Conference (OCC1), September 2009, Cottbus, Germany
[33]. Kranzmann, A., Hünert, D., Rooch, U.I., Schulz, W., Österle, W., 2009. Reactions at the Intereface between Steel and Oxide Scale in wet CO2 Containing Environment. NACE International , Corrosion 2009, 26–26/03/2009, Atlanta, Georgia.
[34]. Abellán, J.P., Olszewski, T., Meier, G.H., Singheiser, L., Quadakkers, W.J., 2010. The oxidation behaviour of the 9% Cr Steel P92 in CO2- and H2O-rich gases relevant to oxy-fuel environments. International Journal of Materials Research 101 (2), 287–299, http://dx.doi.org/10.3139/146.110271
[35]. Otsuka, N., 2013. Carburization of 9% Cr steels in a simulated oxy-fuel corrosion environment. Oxid. Metals 80 (5–6), 565–575, http://dx.doi.org/10.1007/s11085- 013-9396-9
[36]. Kull, R., Maier, J., Mönckert, P., Scheffknecht, G., Hjornhede, A., Anheden, M., 2008. Summary Report on Fly Ash, Deposit, Slagging and Corrosion under Oxy-fuel Conditions in a 500 kW Test Rig. Public Summary Report of the ENCAP Project.
[37]. Gosia Stein-Brzozowska, Jörg Maier, Günter Scheffknecht, (2011), Impact of the oxy-fuel combustion on the corrosion behavior of advanced austenitic superheater materials Gosia Stein-Brzozowska, Jörg Maier, Günter Scheffknecht, Science Direct, https://doi.org/10.1016/j.egypro.2011.02.085.
[38]. Anheden, M., Burchhardt, U., Ecke, H., Faber, R., Jidinger, O., Giering, R., Kass, H., Lysk, S., Ramström, E., Yan, J., 2011. Overview of operational experience and results from test activities in Vattenfall’s 30 MWth oxy-fuel pilot plant in schwarze pumpe. Energy Procedia 4, 941–950, http://dx.doi.org/10.1016/j.egypro.2011. 01.140 (GHGT-10).
[39]. Gopalakrishnan P, Bobba MK, Seitzman JM (2007) Controlling mechanisms for low NOx emissions in a non-premixed stagnation point reverse flow combustor. Proc Combust Inst 31:3401–3408
[40]. Bobba MK, Gopalakrishnan P, Periagaram K, Seitzman JM (2008) Flame structure and stabilization mechanisms in a stagnation-point reverse-flow combustor. J Eng Gas Turbines Power 130:031505-1
[41]. Castela M, Veríssimo AS, Rocha AMA, Costa M (2012) Experimental study of the combustion regimes occurring in a laboratory combustor. Combust Sci Technol 184(2):243–258
[42]. Undapalli S, Srinivasan S, Menon S (2009) LES of premixed and non-premixed combustion in a stagnation point reverse flow combustor. Proc Combust Inst 32:1537–1544
[43]. Luckerath R, Meier W, Aigner M (2008) Flox combustion at high pressure with different fuel compositions. J Eng Gas Turbines Power 130:011505
[44]. Levy Y, Sherbaum V, Arfi P (2004) Basic thermodynamics of Floxcom, the low-NOx gas turbines adiabatic combustor. Appl Therm Eng 24:1593–1605
[45]. Lammel O, Schutz H, Schmitz G, Luckerath R, Stohr M, Noll B, Aigner M, Hase M, Krebs W (2010) Flox combustion at high power density and high flame temperatures. J Eng Gas Turbines Power 132:121503
[46]. Arghode VK, Gupta AK, Bryden KM (2012) High intensity colorless distributed combustion for ultra low emissions and enhanced performance. Appl Energy 92:822–830
[47]. Faqih M, Omar MB, Ibrahim R, Omar BAA. Dry-Low Emission Gas Turbine Technology: Recent Trends and Challenges. Applied Sciences. 2022; 12(21):10922. https://doi.org/10.3390/app122110922B. Rising, letter to U.S. EPA, February 2005.
[48]. Lefebvre AH, Ballal DR (2010) Gas turbine combustion: alternative fuels and emissions, 3rd edn. CRC press, pp 398–414
[49]. L.B. Davis and S.H. Black, “Dry Low NOx Combustion Systems for GE Heavy-Duty Gas Turbines,” GER-3568G: GE Power Systems, October 2000; R. Eldrid, L. Kaufman, and P. Marks, “The 7FB: The Next Evolution of the Gas Turbine,” GER-4194: GE Power Systems April 2001; R.D. Brdar and R.M. Jones, “GE IGCC Technology and Experience with Advanced Gas Turbines,” GER-4207: GE Power Systems, October 2000; F.J. Brooks, “GE Gas Turbine Performance Characteristics,” GER-3567H: GE Power Systems, October 2000; L.B. Davis, “Dry Low NOx Combustion for GE Heavy Duty Gas Turbines,” GER-3568A: GE Power Generation, 1983.
[50]. Zajadatz M, Pennell D, Bernero S, Paikert B, Zoli R, Döbbeling K (2013) Development and implementation of the advanced environmental burner for the Alstom GT13E2. J Eng Gas Turbines Power 135(6):061503
[51]. Zajadatz M, Lachner R, Bernero S, Motz C, Flohr P (2007) Development and design of Alstoms’s staged fuel gas injection EV burner for NOx reduction. In: Proceedings of ASME Turbo Expo 2007 power land, sea air, 14–17 May 2007, Montreal, Canada
[52]. Wind, Torsten & Güthe, Felix & Syed, Khawar. (2015). Co-Firing of Hydrogen and Natural Gases in Lean Premixed Conventional and Reheat Burners (Alstom GT26). 10.1115/GT2014-25813. [Accessed 12. 03. 2024].
[53]. Jansohn, P, Ruck, T, Steinbach, C, Knöpfel, H, Sattelmayer, T, & Troger, C. "Development of the Advanced EV (AEV) Burner for the ABB GTX100 Gas Turbine." Proceedings of the ASME 1997 Turbo Asia Conference. ASME 1997 Turbo Asia Conference. Singapore. September 30–October 2, 1997. V001T05A009. ASME. https://doi.org/10.1115/97-AA-139
[54]. Kedia KS, Ghoniem AF (2012) Mechanisms of stabilization and blowoff of a premixed flame downstream of a heat-conducting perforated plate. Combust Flame 159(3):1055–1069
[55]. Jithin EV, Kishore VR, Varghese RJ (2014) Three-dimensional simulations of steady perforated-plate stabilized propane-air premixed flames. Energy Fuels 28(8):5415–5425
[56]. Altay HM, Park S, Wu D, Wee D, Annaswamy AM, Ghoniem AF (2009) Modeling the dynamic response of a laminar perforated-plate stabilized flame. Proc Combust Inst 32 I(1):1359–1366
[57]. Jamal M, Ibrahim H, Ali M, Elmahallawy M, Abdelhafez A, Nemitallah A, Rashwan S, Habib A (2017) Structure and lean extinction of premixed flames stabilized on conductive perforated plates. Energy Fuels
[58]. Kedia KS, Ghoniem AF (2013) An analytical model for the prediction of the dynamic response of premixed flames stabilized on a heat-conducting perforated plate. Proc Combust Inst 34(1):921–928
[59]. Rashwan SS, Ibrahim AH, Abou-Arab TW, Nemitallah MA, Habib MA (2017) Experimental study of atmospheric partially premixed oxy-combustion flames anchored over a perforated plate burner. Energy 122:159–167
[60]. Edacheri Veetil J, Aravind B, Mohammad A, Kumar S, Velamati RK (2017) Effect of hole pattern on the structure of small-scale perorated plate burner flames. Fuel 216:722–733
[61]. Wang CJ, Wen JX (2014) The effect of a perforated plate on the propagation of laminar hydrogen flames in a channel—a numerical study. Int J Hydrogen Energy 39(36):21335– 21342